The parts of a quantum computer are essential to understanding how this groundbreaking technology is reshaping computation and problem-solving. Quantum computing is an exciting and rapidly evolving field, potentially revolutionizing various industries. Unlike classical computers, which rely on bits as the smallest unit of data, quantum computers use qubits. Thanks to the principles of quantum mechanics, these qubits can exist in multiple states simultaneously. This unique feature allows quantum computers to process and store information far more efficiently than classical systems, enabling them to solve complex problems that would be impossible or take an impractically long time on traditional computers.
- Understanding Quantum Computing
- Core Components/Parts of a Quantum Computer
- Qubits
- Quantum Gates
- Quantum Processor (Quantum Chip)
- Control Electronics
- Measurement Devices
- Supporting Systems
- Recent Innovations in Quantum Computing
- Applications Across Industries
- Challenges Facing Quantum Computing
- The Future Landscape
- Conclusion
This comprehensive guide will delve deep into the various parts of a quantum computer, examining their roles, functionality, and recent advancements. From qubits and quantum gates to the cryogenic systems required for their operation, each component plays a vital role in making quantum computing possible. Understanding these parts of a quantum computer is crucial for appreciating its full potential and the impact it is likely to have across numerous sectors, including cryptography, healthcare, and materials science. Let’s explore these components in detail to see how they collectively power the quantum revolution.
Understanding Quantum Computing
Before diving into the specific parts of a quantum computer, it is essential to understand what quantum computing is and how it differs from classical computing.
Definition:
Quantum computing harnesses the principles of quantum mechanics to perform calculations that would be impossible or take an impractical amount of time for classical computers.
Key Principles:
The two fundamental principles that underpin quantum computing are superposition and entanglement.
- Superposition allows qubits to represent both 0 and 1 at the same time. This ability allows quantum computers to execute numerous calculations at the same time.
- Entanglement refers to a phenomenon in which qubits become linked, allowing the state of one qubit to be influenced by the state of another, regardless of the distance between them. This property can lead to faster processing speeds and more efficient computations.
Core Components/Parts of a Quantum Computer
The parts of a quantum computer can be categorized into several key components:
- Qubits
- Quantum Gates
- Quantum Processor (Quantum Chip)
- Control Electronics
- Measurement Devices
- Classical Computer Interface
- Cryogenic Systems
- Quantum Error Correction Systems
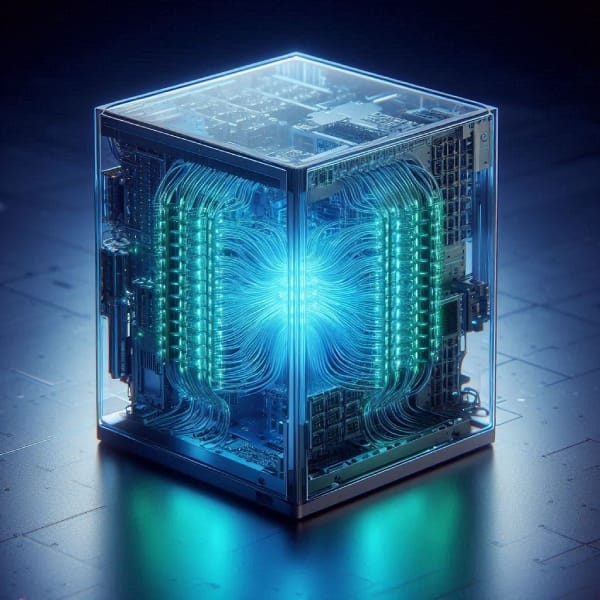
Qubits
Qubits are the fundamental units of information in a quantum computer. They differ from classical bits in several ways:
Types of Qubits:
There are several implementations for qubits, each with unique characteristics:
- Superconducting Qubits: These qubits are made from superconducting materials like niobium or aluminum. They operate at extremely low temperatures and are among the most widely used types in current quantum processors.
- Trapped Ion Qubits: In this approach, individual ions are confined using electromagnetic fields and manipulated with lasers. Trapped ion systems have demonstrated high fidelity and long coherence times.
- Photonic Qubits: Utilizing photons as qubits allows for room-temperature operation and integration with existing optical technologies. Photonic qubits are particularly promising for quantum communication applications.
Each type of qubit has its advantages and challenges, contributing to ongoing research aimed at improving its performance and scalability.
Quantum Gates
Quantum gates are essential components that manipulate qubits through various operations. They serve as the building blocks for quantum circuits, similar to logic gates in classical computing, highlighting the importance of the parts of a quantum computer in performing complex calculations. These are the types of Quantum Gates:
- Hadamard Gate: This gate creates superposition by transforming a single qubit into an equal probability state between 0 and 1.
- CNOT Gate (Controlled NOT): The CNOT gate flips the state of one qubit based on another’s state, enabling entanglement between qubits.
- Pauli Gates: These gates include X (NOT), Y, and Z gates, which perform specific transformations on qubit states.
Quantum gates enable complex operations necessary for executing quantum algorithms, making them indispensable in the design of quantum circuits.
Quantum Processor (Quantum Chip)
The quantum processor is often referred to as the “brain” of the quantum computer. It houses multiple qubits and is responsible for executing quantum algorithms. The architecture of these chips is critical for enhancing performance and scalability:
- Design Considerations: Researchers focus on optimizing qubit connectivity, minimizing crosstalk between qubits, and improving coherence times to enhance overall computational power.
- Scalability: As technology advances, there is a push towards developing processors capable of housing thousands or even millions of qubits, significantly increasing computational capabilities.
Recent advancements have led to breakthroughs in chip design that allow for more efficient operations while maintaining stability under operational conditions.
Control Electronics
Control electronics play a vital role in managing the operations of qubits. These systems generate microwave pulses that manipulate qubit states with high precision:
- Signal Generation: Control electronics must produce accurate signals that correspond with specific operations required by the quantum gates.
- Noise Mitigation: Minimizing noise introduced during signal transmission is crucial for maintaining qubit coherence and ensuring reliable computations.
Innovations in control electronics continue to improve the fidelity and efficiency of operations within quantum systems.
Measurement Devices
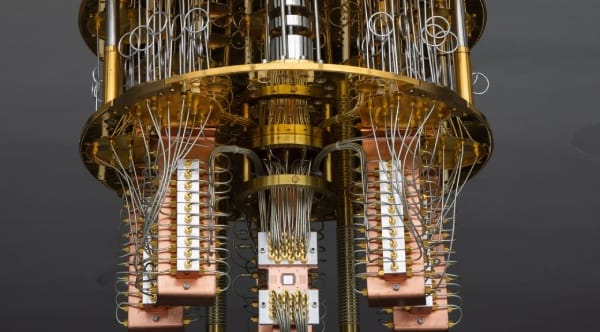
Once computations are completed, measurement devices extract information from qubits. This process collapses the superposition state into a definite value (either 0 or 1), providing the output of the computation:
- Measurement Techniques: Various measurement techniques exist, including projective measurement and weak measurement, each with different implications for accuracy and disturbance to the system.
- Importance of Accuracy: Accurate measurement is essential for ensuring reliable results from quantum algorithms, particularly as systems grow more complex with increased numbers of qubits.
Supporting Systems
In addition to core components, several supporting systems enhance the functionality and reliability of quantum computers:
Classical Computer Interface
Quantum computers require classical systems for interfacing with users. These classical computers handle tasks such as programming the quantum computer, sending it instructions, and processing results:
- Hybrid Approach: This hybrid approach combines classical computing’s strengths with those of quantum computing, allowing users to leverage both technologies effectively.
Cryogenic Systems
Many types of qubits require extremely low temperatures to function correctly. Cryogenic systems cool down the quantum processor to near absolute zero:
- Dilution Refrigerators: These advanced cooling systems maintain low temperatures necessary for superconducting qubits while minimizing thermal noise that could interfere with operations.
Maintaining optimal operating conditions through cryogenic systems is essential for achieving high coherence times in qubits.
Quantum Error Correction Systems
Error correction is vital in quantum computing due to the fragile nature of qubits. Quantum error correction codes help detect and correct errors that may occur during computations:
- Error Correction Techniques: Techniques such as surface codes have shown promise in reducing error rates by encoding logical qubits into multiple physical qubits, thereby enhancing fault tolerance.
Implementing effective error correction methods is crucial for improving reliability in practical applications involving large-scale quantum computations, especially when considering the different parts of a quantum computer.
Recent Innovations in Quantum Computing
As we explore advancements in 2024, several innovations are reshaping our understanding of the parts of a quantum computer:
- Researchers are developing new materials aimed at improving existing components or creating entirely new types of qubits that enhance performance while reducing size and complexity.
- Advances in AI integration into quantum computing systems are optimizing algorithms and enhancing error correction capabilities further solidifying the relationship between these two fields.
- The concept of a quantum internet is gaining traction, with progress in quantum key distribution (QKD) protocols promising secure communication channels based on principles derived from quantum mechanics.
Applications Across Industries
The potential applications for quantum computing span numerous industries, each leveraging different parts of a quantum computer to solve complex problems.
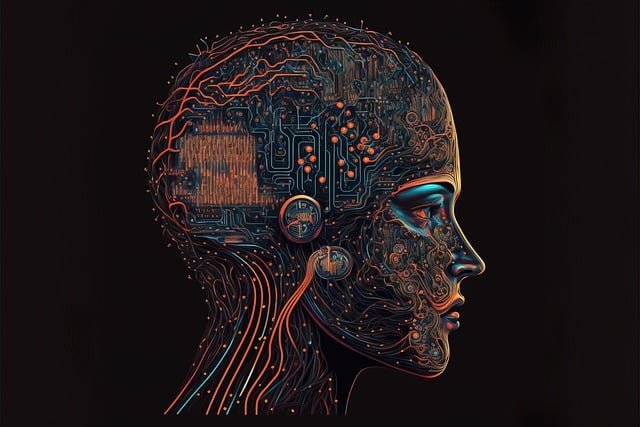
- In cryptography, developing algorithms resistant to attacks from future powerful quantum machines will safeguard sensitive data against emerging threats.
- The financial services sector stands to benefit from improved modeling techniques that enhance risk management strategies through faster processing capabilities offered by quantum computers.
- In pharmaceuticals, accelerated drug discovery processes could lead to breakthroughs in treatment options through simulations that model complex molecular interactions more efficiently than traditional methods allow, utilizing various parts of a quantum computer to achieve these advancements.
- Materials science will leverage advancements in quantum computing for designing new materials with tailored properties impacting industries ranging from electronics to renewable energy solutions.
Challenges Facing Quantum Computing
Despite its potential, several challenges must be addressed before widespread adoption becomes feasible:
Scalability Issues
Scaling up from small-scale prototypes to large-scale operational systems poses significant technical hurdles. Ensuring that all parts of a quantum computer work harmoniously together becomes increasingly complex as more qubits are added to a system.
Error Rates
Current error rates remain higher than desirable levels due to environmental noise affecting delicate qubit states. Continued research into error correction techniques for the parts of a quantum computer will be crucial for making practical applications viable across various sectors.
Resource Requirements
The need for advanced materials combined with sophisticated cooling technologies makes building functional large-scale systems resource-intensive both financially and logistically, as these innovations must support the intricate parts of a quantum computer.
The Future Landscape
As we look ahead at what lies beyond today’s developments within this fascinating field, mastering these parts of a quantum computer will unlock capabilities that promise transformative impacts across diverse domains—from cryptography and finance to healthcare. Quantum computing’s enhanced computational power will address pressing global challenges like climate change through innovative solutions. If you’re interested in the cutting-edge technology behind big data and machine learning, check out our guide on the 7 best laptops for big data and machine learning here, where we explore powerful tools for handling complex computations.
Conclusion
In conclusion, understanding the parts of a quantum computer is crucial for recognizing its potential to revolutionize multiple sectors. Each part plays a vital role in harnessing the unique properties derived from the laws of the universe, enabling quantum computers to solve complex problems that traditional methods cannot effectively tackle.
As research and technological advancements continue rapidly, innovations emerge within this dynamic field. The synergy between AI, software development, and hardware innovation is positioning quantum computing to go mainstream, unlocking new frontier discovery and problem-solving that were previously unimaginable, particularly in understanding the parts of a quantum computer and its applications.
The journey to understanding these intricate and beautifully designed parts of a quantum computer is just beginning, yet the implications are already profound and far-reaching. By delving deeper into each part’s function and significance, we can better appreciate how they work together harmoniously, creating powerful computational tools that promise to reshape society in ways we are only just starting to envision.